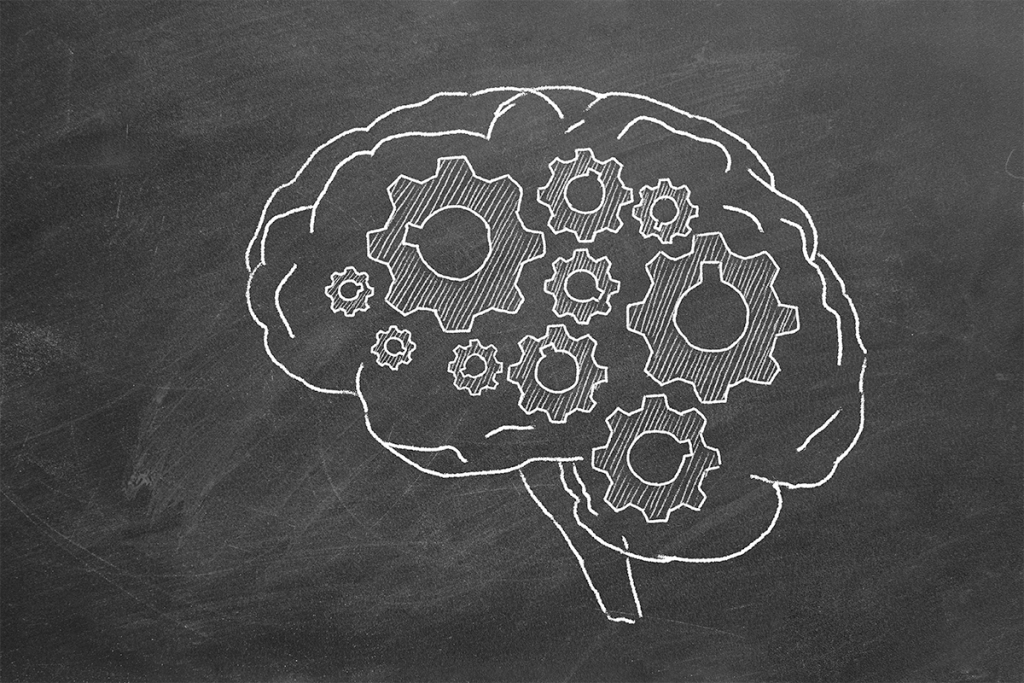
It’s easy to get lost when motoring down the epigenetic landscape; thankfully, a group of cunning cartographers from the Brain Research through Advancing Innovative Neurotechnologies Initiative Cell Census Network (or BICCN for short) has generated single-cell maps to guide your voyage of the brains’ motor cortex. Although this epigenetic road trip may be too long for us to tackle in-depth, here we describe the landmarks for you to check out on your voyage through this recently published trove of nearly thirty publications that offer multi-omic single-cell data from humans, mice, and monkeys. But maybe start your downloads now, since this one takes up about 241 terabytes of storage!
Integrated Epigenomic and Transcriptomic Maps Detail the Mouse Motor Cortex
In their study, researchers led by Eran A. Mukamel (University of California, San Diego) generated single-cell transcriptomic and DNA methylation/chromatin accessibility maps for adult mouse primary motor cortex neurons. Rather than deriving multimodal data from single cells, the authors employed two computational methods (SingleCellFusion and LIGER) to integrate multiple datasets from single-cell (sc) and single-nucleus (sn) RNA-sequencing, single-nucleus DNA methylation sequencing (snmC-seq2), and single-nucleus assay for transposase-accessible chromatin using sequencing (snATAC-seq) experiments without losing resolution.
This data provided insight into the molecular architecture of primary motor cortex cell types and identified networks of gene expression regulatory elements marked by overlapping regions of open chromatin and cell-type-specific DNA demethylation. Interestingly, the cross-validation and multimodal integration supported the existence of “clusters” spanning 30 to 116 cell types; therefore, the authors suggest that motor cortex cells exist across a molecular continuum and advise against “pigeonholing” cells into discrete categories.
SnapATAC Maps Gene Regulatory Regions of Mouse Brain
Researchers led by Bing Ren (University of California, San Diego) employed single-cell combinatorial indexing (sci)ATAC-seq to explore the active transcriptional regulatory elements present in 160 distinct cell types derived from 45 adult mouse brain regions, including the motor cortex. The integration of scRNA-seq data in this study provided further insight into the gene regulatory programs active in the mammalian brain while also driving home our understanding of the links between non-coding risk variants and neurological conditions in human patients.
The authors also introduced a new technique -SnapATAC – as a significantly improved means of analyzing scATAC-seq datasets (as described in an accompanying paper) and demonstrated its potential by describing the discovery of over 370,000 transcriptional regulatory elements in over thirty distinct cell populations from the mouse secondary motor cortex and inferring cell-type-specific transcriptional regulators.
Mouse Brain DNA Methylation the Provide the Basis for a Neural Network Model
In their DNA methylation-centric study, researchers led by Joseph R. Ecker (Salk Institute for Biological Studies) profiled the DNA methylome of the brain region-specific cell types identified in the previous study using snmC-seq2. Moreover, the authors performed single-nucleus methylation and chromosome conformation capture sequencing (sn-m3C-seq) and integrated this data with aforementioned snATAC-seq data to fully comprehend the existing cell-type-specific regulatory elements, candidate target genes, and upstream transcription factors.
Overall, the authors identified nearly four million regions with cell-specific DNA methylation patterns; excitingly, the differential methylation patterns of transcription factor genes and binding motifs allowed a prediction of lineage-specific and cell-type gene regulatory programs. Integrating snATAC-seq data enabled the prediction of enhancer-like genomic regions, while sn-m3C-seq identified cis-regulatory chromatin contacts between enhancers and genes in the hippocampus. Most interestingly, the study used methylome profiles as an input for an artificial neural network model that could predict the identity and location of single cells in the brain.
Epi-retro-seq Describes the Diversity of Mouse Cortical Projection Neurons
In their “up-to-date” study, researchers led by Edward M. Callaway (Salk Institute for Biological Studies) employed epi-retro-seq, which applies snmC-seq to neurons dissected from cortical regions (including the motor cortex) labeled based on their cortical and subcortical projection, to identify potential regulatory elements and predict gene expression in the same neuron. The authors employed this approach to better understand any relationship between the molecular and anatomical features defining cortical neurons.
Fascinatingly, their analysis of nearly 12,000 single neocortical neurons provided evidence that their diverse DNA methylation landscapes correspond to their cortical and subcortical location and projection patterns. Furthermore, profiling cell-specific differentially methylated regions allowed the authors to identify putative transcription factors linked to different projection cell types, which provides insight into the epigenetic mechanisms contributing to morphological and functional differences.
Human Cortical Development as Described by Single-cell Epigenomics
A team led by Tomasz J. Nowakowski (University of California, San Francisco) took their mapping efforts to the developing human brain, where they applied scATAC-seq to primary human forebrain tissue (including the motor cortex). They aimed to identify regions undergoing cell type- and brain region-specific changes in accessibility during neurogenesis and cell-type-specific candidate regulatory elements. A fascinating comparison of in vivo patterns to those of cerebral organoids generated via the three-dimensional differentiation and culture of induced pluripotent stem cells revealed that organoids recapitulated cell-type-specific enhancer accessibility patterns well (even though they failed to faithfully mirror all open chromatin regions found in vivo).
Overall, this study highlighted putative regulatory mechanisms controlling neurogenesis, linked the chromatin landscape with disease variants in developing cortical neurons to neuropsychiatric disorders, and confirmed the already established role of retinoic acid signaling in cortical arealization during forebrain development. Furthermore, the team provided evidence for the robust recapitulation of neural development in stem cell-derived cerebral organoids, supporting the relevance of ongoing studies using these vitally important model systems.
The Motor Cortex – Of Mice and Men……..and Monkeys!
A final study from researchers led by Trygve E. Bakken and Ed S. Lein (Allen Institute for Brain Science, Seattle) took motor cortex analysis beyond that of mice and men to also include monkeys (marmosets and macaques, to be precise)! Their study of both evolutionary conservation and divergence evaluated the neural and non-neural cellular composition of the motor cortex through single-nucleus transcriptomic assays and epigenomic assays that included snmC-seq2 and single-nucleus chromatin accessibility and messenger RNA expression sequencing (SNARE–seq2), a technique developed by the lab of Kun Zhang (University of California, San Diego) and described in great detail in a related article.
While the resultant data provided evidence of cross-species conservation, the authors also noted species-dependent specializations in cell proportions, gene expression, DNA methylation, and chromatin state. Interestingly, few marker genes displayed conservation in specific cells; however, this provided a concise list of genes and regulatory mechanisms that may support the conserved features of homologous cell types. Finally, the authors also described how the combination of whole-cell patch-clamp recordings, RNA sequencing, and morphological characterization – a technique known as “patch-seq” – helped characterize the specialized physiology and anatomy of specific corticospinal cells in monkeys and humans.