Gene drives, which could theoretically engineer entire species, are a much-ballyhooed but little tested application for CRISPR-based genetic engineering. Gene drives would bias inheritance in sexual reproduction, with drive alleles passing themselves on nearly 100% of the time, instead of the 50% for normal Mendelian inheritance. Including a CRISPR effector like Cas9, a guide RNA, and an optional “cargo” gene, the drive cuts the target gene and copies itself in the target’s place.
Gene drive ballyhooing includes such promises as malaria-proof mosquitoes, but the lack of testing stems from fears of escaped drives spreading unchecked. One recommended way to make gene drives safer is to put the cas9 gene in a second site, so the drive can only spread in test populations that already express Cas9. Such a split drive would be safe, but not useful for real-world implementation, because it wouldn’t be able to spread in wild populations.
This presents a conundrum: should gene drive tests be effective or safe? However, as we know from other intractable problems – from beautifying highways to forgetting your anniversary to deciding whether or not you like butter – flowers are pretty much always a good solution. Working under this assumption of floral amelioration, Charleston Noble and John Min, with gene drive pioneer Kevin Esvelt at Harvard and MIT, propose a daisy-chain gene drive. Daisy drives would solve the safety vs efficacy trade-off by spreading in wild populations, but only for a limited time and in a limited space.
Daisy Drive: Beautification Of The Gene Drive
The idea behind daisy drives is to create a cascade of drive alleles daisy-chained together. No allele would drive itself; instead, each would drive the next in the chain. The top of the daisy chain would be a “payload” including cas9 but no guide RNA. Below that would be an arbitrary number of daisy links in different loci, each including a guide RNA targeting the wild-type version of the next-higher daisy in the chain. Since the bottom daisy in the chain has no guide RNA driving it, it would eventually be lost. That would leave the next daisy with nothing driving it, so that would eventually be lost, and so on.
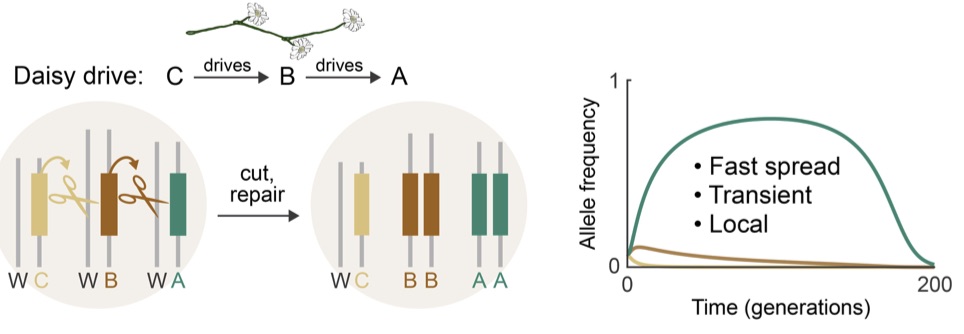
In a daisy chain gene drive, each allele drives the next higher in the chain. The current bottom allele is not driven, so natural selection eventually chews away the daisy chain from the bottom up. (Image via Noble et al. http://dx.doi.org/10.1101/057307)
The authors only tested the daisy drive in simulations, but they find that even three-link chains could peak above 99% of a population with an initial release at only 5% of the population size. Longer chains could spread with lower release sizes, but higher fitness costs or lower homologous recombination rates would reduce the spread.
Orthogonal Guide RNAs For Optimal Daisy Arrangement
Daisy drives are a pretty idea, but as any florist will tell you, the arrangement is key. In this case, the daisy elements would have to stay in a linear chain, with no recombination. Recombination would be bad for two reasons: first, it could break the chain, or second, it could tie the chain into a “necklace” – i.e., A drives B drives C drives A – that could spread unconstrained, like a “classical” drive.
To prevent recombination and maintain the chain arrangement, the authors developed a set of maximally orthogonal guide RNAs that still work with Cas9 and tested them experimentally. Combined with different promoters, these should minimize recombination between drive elements. Beyond daisy drives, these recombination-resistant guide RNAs could also improve synthesis and stability of multiplex targeting arrays for traditional CRISPR-Cas9 gene editing.
Gene drives are not likely to be tested in the wild any time soon, the FDA’s recent approval of field tests for population-suppressing GM mosquitos notwithstanding. With their inherent safety combined with low required release numbers, daisy drives seem much more promising for real-world testing.
For more daisy arrangement tips and a free vase (maybe unlikely, but who knows where this preprint will eventually come out?) check out the preprint and/or published paper from Charles Noble, John Min, et al., 2016.